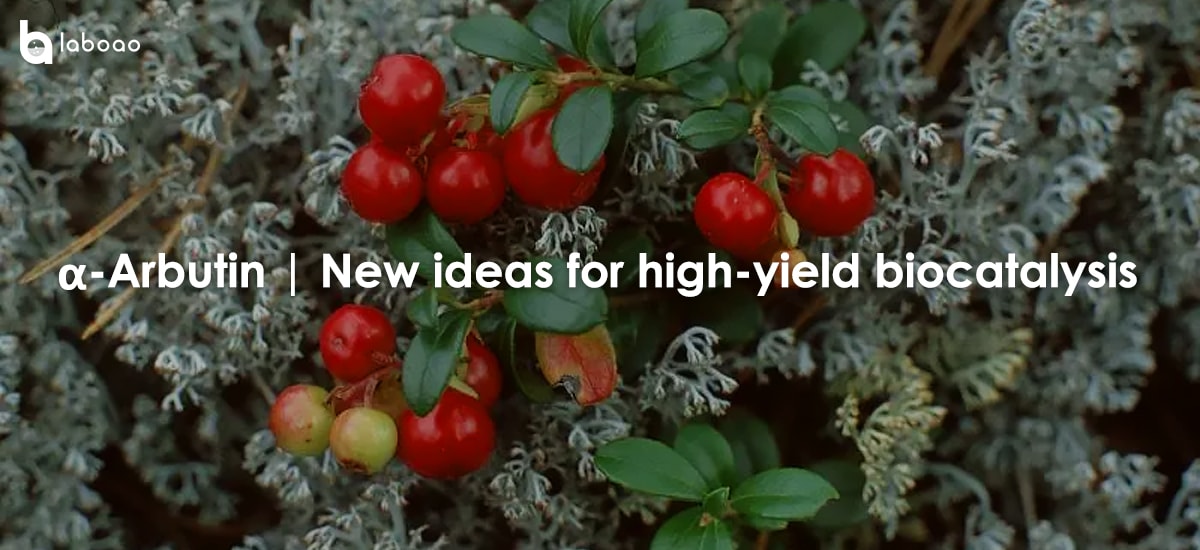
Professor Liu Long’s team from Jiangnan University’s Key Laboratory of Glycochemistry and Biotechnology of the Ministry of Education published an article in "Applied microbiology and biotechnology": "Combinatorial metabolic engineering enables high yield production of α-arbutin from sucrose by biocatalysis", through knockout and sucrose hydrolysis Genes related to the enzyme increase the sucrose conversion rate to 51.5%. An inducible protein degradation system was also developed, using Lon protease and proteolytic tag to control the activity of PfkA, thereby converting more fructose-6-phosphate into glucose-1-phosphate for α-arbutin synthesis.
And by integrating another copy of 6-phosphate glucose isomerase and phosphate glucose decomposing enzyme, the pathway from F6P to Glc1P was strengthened, a high α-arbutin titer was obtained, and the sucrose conversion rate was increased to 60.4%.
In this study, substrate utilization was improved due to the attenuation of hydrolysis and the assistance of intracellular enzymes that reconvert the by-products into substrates for α-arbutin synthesis. This strategy provides a new idea for whole-cell biocatalytic synthesis of other products using sucrose as a substrate, especially valuable sugars.
α-Arbutin has previously been produced by chemical synthesis and biotransformation. Among them, the chemical synthesis of α-arbutin requires the protection and deprotection of the OH group due to the harsh reaction conditions and complex reaction process. In contrast, biotransformation of α-arbutin was considered as a potential candidate. Because its synthesis procedures are simpler, the reaction conditions are mild, and there is less pollution, including enzymatic conversion, microbial fermentation, and whole-cell biocatalysis.
Whole-cell biocatalysis of α-arbutin was performed by engineered microbial cells expressing glycosyltransferases from different sources. They all use hydroquinone as the acceptor substrate, whose phenolic hydroxyl group can form α-isomeric glycosidic bonds with sugar moieties from different donor substrates. Hydroquinone is easily oxidized under light to form dark-colored benzoquinone that has no ability to accept glycosides, and then generates oxygen free radicals, inhibiting the catalytic activity of the enzyme.
Whole-cell biocatalysis has several advantages: simple cell post-processing, high synthetic yields, and simple product isolation and purification. In addition, whole-cell catalysts can be recovered by simple centrifugation, thereby reducing industrial production costs. However, in the previous whole-cell biocatalytic production of arbutin, the conversion rate of sucrose to α-arbutin was very low.
Figure 1. Operations related to sucrose metabolism and α-arbutin synthesis program by Bacillus subtilis.
Block the extracellular hydrolysis pathway of sucrose
During the growth of Bacillus subtilis, the dual-region sacB-levB transcript and its products levansucrase and endolevanase are involved in extracellular sucrose metabolism in a certain order. Extracellular sucrose can induce the production of secreted levansucrase and membrane-anchored endolevanase, which then degrade sucrose into glucose and fructose outside the cell. Neither is a suitable donor substrate in the biotransformation of α-arbutin. Therefore, this part of the added sucrose is wasted.
In order to avoid the extracellular breakdown of sucrose, the sacB, sacC, and levB genes were knocked out to generate recombinant strains BS8S401, BS8S402, and BS8S403, respectively (Fig. 2A). Knocking out the sacB, sacC, and levB genes had no obvious effect on cell growth (Fig. 2B), whereas BS8S4 depleted sucrose within 24 h (Fig. 2C). The results show that knocking out genes related to sucrose extracellular metabolism can significantly reduce sucrose consumption during cell growth.
Figure 2. Effects of deletion of important genes in the extracellular sucrose decomposition pathway on α-arbutin production and sucrose consumption of recombinant Bacillus subtilis strains. A: Schematic diagram of knockout of sacB, sacC and levB genes. BS8S401 was obtained by deleting the sacB gene in BS8S4; this strain was used as the starting strain for expressing Smut in Bacillus subtilis WB800. Similarly, BS8S402 was obtained by deleting the sacC gene in BS8S401, and BS8S403 was obtained by deleting the levB gene in BS8S402. B: Cell growth curve of the recombinant strain. The vertical axis is the sucrose concentration remaining in the culture medium. C: Comparison of sucrose utilization abilities of different recombinant strains. D-F: Comparison of α-arbutin titers and sucrose consumption of different recombinant strains.
Block the intracellular competition pathway for sucrose
Sucrose is transported into cells via the PTS pathway and converted into sucrose-6-phosphate. The sacA-encoded sucrose-6-phosphate hydrolase competes with Smut for the same substrate (sucrose-6-phosphate).
In order to improve the conversion rate of sucrose-6-phosphate to α-arbutin, the sacA gene of BS8S401 was knocked out, thus forming the recombinant strain BS8S404 (Fig. 3A).
Figure 3. Effect of sacA gene deletion on α-arbutin biotransformation. A: BS8S404 is obtained by deleting the sacA gene of BS8S401. B: Comparison of sucrose conversion rates of different recombinant Bacillus subtilis. C: Comparison of consumption rates of different recombinant Bacillus subtilis.
BS8S401 and BS8S404 were cultured for whole-cell biocatalytic preparation. The experimental results showed that the deletion of the sacA gene did not interfere with cell growth. During whole-cell bioassays, sucrose consumption in BS8S404 cells was reduced by 17.4% over 24 hours compared to BS8S401 (Figure 3B), indicating that inactivation of SacA prevents the hydroxylation of sucrose-6-phosphate, forming additional sucrose in α-Arbutin is consumed in bioanalysis. However, within 24-48 hours, the sucrose consumption rate of BS8S404 cells gradually increased and approached that of BS8S401 cells, and the sucrose conversion rate finally reached 51.5%.
Regulation of pfkA expression through protein degradation tags
As a by-product of α-arbutin synthesis by engineered Bacillus subtilis, fructose can be converted into G1P, another glycosyl donor substrate of Smut, to increase the carbon atom conversion efficiency of α-arbutin sucrose, aiming to reduce phosphoric acid fructokinase activity to save more F6P for α-arbutin synthesis (Fig. 1B).
Bacillus subtilis can effectively utilize glycerol as an ideal carbon source to generate intermediate glyceraldehyde 3-phosphate, which then bypasses PfkA and enters the downstream glycolysis and TCA cycle (Figure 4A). Therefore, the pfkA gene was knocked out and the recombinant strain BS8S405 was generated. The growth of BS8S405 was significantly inhibited.
Figure 4. Glycerol utilization pathway of Bacillus subtilis and engineering strategies for tunable PfkA degradation.
In addition, the researchers used a protein degradation tag strategy to regulate the expression and degradation of PfkA (Figure 4B) to ensure sufficient cellular biomass. This system consists of a peptide tag fused to the C-terminus of PfkA and xylose-induced MfLon. Therefore, constitutively expressed PfkA can be degraded in a PDT-dependent manner.
The recombinant strain BS8S406 was constructed by inserting the mfon gene into the genome of BS8S404, and this strain can express MfLon by adding xylose. The CRISPR/Cpf1 system was used to fuse candidate PDTs to the C-terminus of PfkA. Four plasmids were constructed, each constituting BS8S406. Finally, the recombinant strains BS8S406-PWT and BS8S406-P3 were obtained (Fig. 4B).
Summarize
In this study, the researchers developed BS8S407 for whole-cell biocatalysis of α-arbutin with high efficiency by adjusting the native utilization pathway of sucrose and repurposing the by-product for α-arbutin production. Sucrose conversion rate.
Our results demonstrate that combinatorial engineering of exogenous catalytic enzymes and host cell endogenous metabolic pathways can serve as an effective and prospective strategy for whole-cell biocatalysis to improve substrate conversion rates. However, there are still some shortcomings such as sucrose consumption proposed in the later stages of biocatalysis.
In the future, scientists can further try to delete YugT as it is hypothesized to act as an α-glycosidase to prevent sucrose-6-phosphate hydrolysis during whole-cell biocatalysis. In addition, the expression levels of pgi and pgcA can be further increased by inserting more copies, and the adaptability of Smut to Glc1P can also be improved through enzyme modification strategies to make the conversion of F6P to Glc1P more complete. Finally, the promoter for xylose-induced mfon gene expression can be replaced by a period-specific promoter or an auto-inducible promoter to facilitate industrial applications.